Rheology
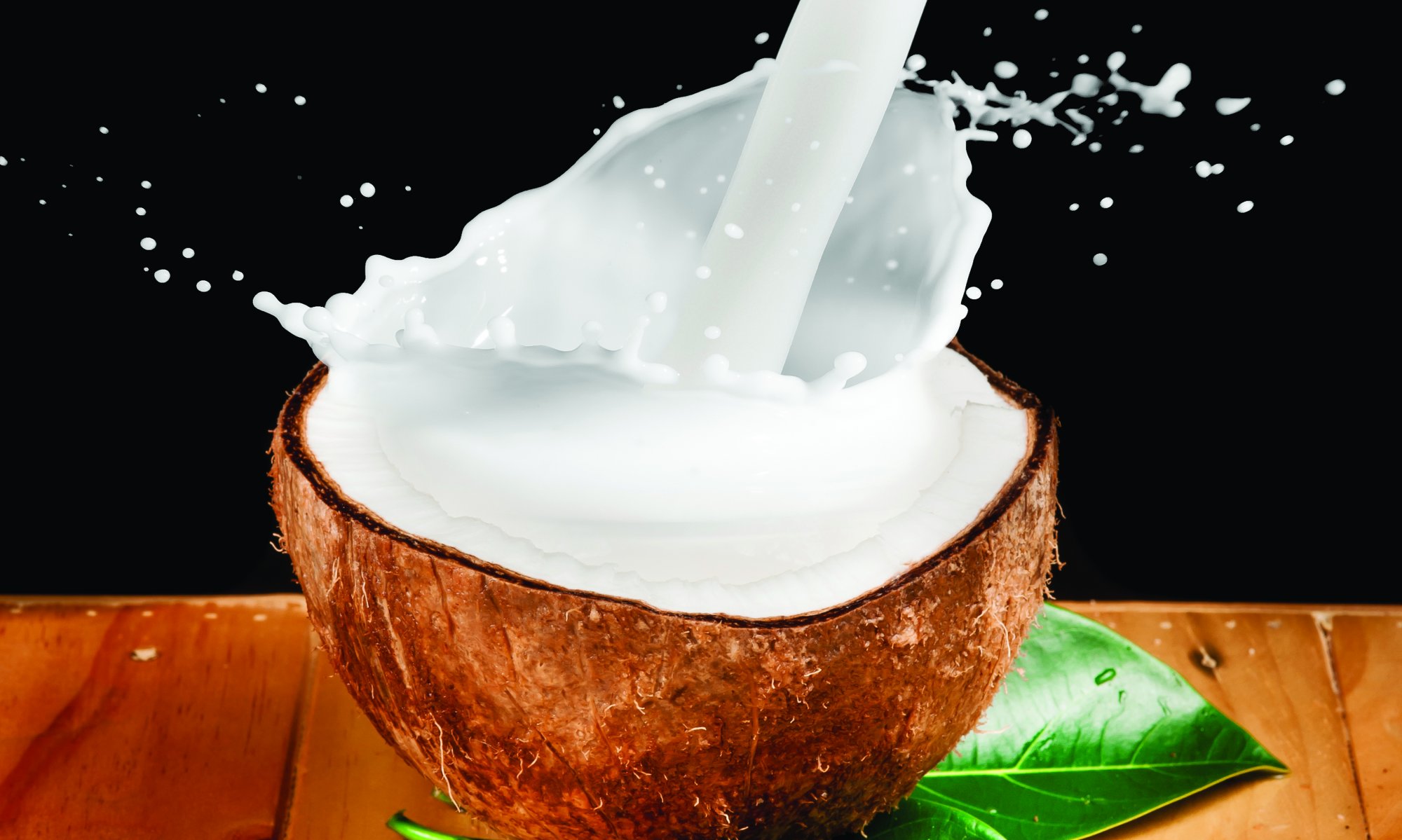
Rheology is one of the most important considerations in designing food processing plants for clarified liquid coconut milk extract. It is defined as the science of dealing with flow, and the deformations that result from flow. Specifically, the deformation of flow involves viscosity – the internal friction which occurs when a layer of fluid is made to move against another layer of fluid.
Shearing
In rheology, shearing is the key to understanding flow behaviour and structure. Shearing between parallel planes is normally used for the basic definition of shear stress and rate, depending on how much and how quickly the deformation is applied to the material (Figure 10.1).
To derive viscosity, shearing must induce the stationary flow of substance. This is achieved by rearranging and deforming particles, breaking the bonds in the structure of the material. For further analysis, shearing is done gently to prevent the structure from destruction. To do so, an oscillating shear, with amplitude low enough to study an unbroken structure, is applied to the material.
A sheared flow occurs between parallel planes. Other flows include a rotational flow between stationary and rotating coaxial cylinders; a telescopic flow through capillaries and pipes and a torsional flow between parallel planes (Figure 10.2).
Types of viscosity
Types of flow
Depending on the material’s flow behaviour, viscosity is classified as Newtonian or non-Newtonian.
Newtonian flow is characterized by viscosity that is independent of the shear rate at which it is measured. There is a constant viscosity dependent on temperature, but independent of the applied
shear rate.
The proportionality constant is equal to the viscosity of the material. The flow curve, which plots shear stress against shear rate, is a straight line with slope η for a Newtonian fluid (Figure 10.3).
On the other hand, the viscosity curve, which plots viscosity against shear rate, will show a straight line at a constant value equal to η (Figure 10.4).
Examples of Newtonian fluids are water, mineral oils, liquid
lecithin, prune concentrate, various syrups, and fructose in water, cottonseed oil, wine and pure sucrose solutions. It also includes low concentration liquids in general, such as whole milk and skim milk.
Non-Newtonian flow is used to describe materials which cannot
be defined by a single viscosity value at a specified temperature.
As such, the viscosity of these materials must always be stated together with its corresponding temperature and shear rate. If the shear rate is changed, the viscosity will also change. In general, high concentration and low temperature may induce or increase non- Newtonian behaviour.
Besides the magnitude of the shear rate, the viscosity of non-Newtonian fluids may also be time dependent. In most cases, the frequency of successive applications of shear also determines viscosity.
Examples of non-Newtonian materials which are dependent on time are yogurt and mayonnaise. Non-Newtonian materials can also be independent of time. Examples are tomato ketchup and coconut milk. For time-independent Non-Newtonian materials, flow behaviour can be classified as shear-thinning, shear-thickening and plastic. While materials that are time dependent are defined as thixotropic, rheopectic or anti-thixotropic.
Shear-thinning flow behaviour
Shear thinning flow behaviour is mainly caused by the increased shear rate deforming and/or re-arranging particles, resulting in lower flow resistance and subsequently, lower viscosity. The viscosity of a shear thinning or pseudo-plastic fluid decreases as shear rate increases. While many liquid food systems belong to this category of fluids, the shear rate dependency of viscosity can vary depending on the type of products, temperatures and concentrations of a given liquid.
For coconut milk, studies have shown that it exhibits shear thinning behaviour over 15-30% oil concentrations when mixed with emulsifiers, pre-heated and homogenized (Tipvarakarnkoon, 2009).
Shear-thickening flow behaviour
A shear thickening fluid usually exhibits dilatant flow behaviour. This is generally found among very high concentration suspensions. The viscosity of a shear thickening fluid increases as shear rate increases. The solvent acts as a lubricant between suspended particles at low shear rates, but is squeezed out at higher shear rates, resulting in denser packing of particles.
Plastic flow behaviour
A plastic fluid exhibits yield stress. The result is that a significant force must be applied before the material starts to flow like a liquid, which is often referred to as the ketchup effect. If the force applied is smaller than the yield stress, the material stores the deformation energy and exhibits elastic properties while behaving like a solid. The liquid starts to flow like a Newtonian liquid when yield stress is exceeded. Otherwise known as a Bingham plastic liquid, it can also flow like a shear thinning, visco-plastic liquid.
Typical plastic fluids are quark, tomato paste, certain ketchups and greases. For time-dependent Non-Newtonian materials, flow behaviour can be classified as thixotropic, anti-thixotropic and rheopectic.
Thixotropic flow behaviour is normally studied in a loop test when the material is subjected to increasing shear rates, followed by the same shear rates in decreasing order. A thixotropic fluid is a shear thinning system where viscosity decreases with a constantly increasing shear rate. It also decreases with time at a constant shear rate.
Time-dependent thixotropic flow behaviour is observed from the difference between ascending and descending viscosity and shear stress curves (Figure 10.5).
To recover its structure, the material must rest for a certain period of time, which is characteristic of those included in gel-forming systems. Typical examples are yoghurt, mayonnaise, margarine, ice cream and brush paint.
Rheopectic flow behaviour
A rheopectic fluid is a thixotropic fluid with a structure that only recovers completely if subjected to a small shear rate. This means that a rheopectic fluid will not rebuild its structure at rest.
Anti-thixotropic flow behaviour
Anti-thixotropic flow behaviour is also illustrated by a loop test and is very uncommon among foodstuffs. This is because anti-thixotropic fluids have a shear thickening system where viscosity increases not only with increasing shear rate, but also with time at a constant shear rate.
Several models are available to mathematically describe the flow behaviour of non-Newtonian systems. Examples are Ostwald, Herschel-Bulkley, Steiger-Ory, Bingham, Ellis and Eyring. These models relate the shear stress of a fluid to the shear rate. As always, the apparent viscosity is calculated as the variable between shear stress and shear rate.
Flow behaviour models
Power law equation
The most general flow behaviour model is the Herschel-Bulkley model, also known as the power law equation. In principle, it is an extended Ostwald model. This equation is applicable to a great number of non-Newtonian fluids over a wide range of shear rates. It is also applicable to plastic, shear thinning and shear thickening fluids according to
The power law equation also lends itself readily to calculating pressure drop and heat transfer. Thus, it is the most appropriate rheological model for clarified liquid coconut milk extract. The apparent viscosity of clarified liquid coconut milk extract decreases when temperature increases. When concentration of clarified liquid coconut milk extract increased, the apparent viscosity also increased (Simuang et al., 2004).
Appropriate modifications of the generalized power law equation make it possible to express each type of flow behaviour.
For Newtonian fluids, the power law equation, given K = η and n = 1, is specified as
For a plastic fluid, the power law equation is used in the fully generalized form, with n<1 for visco-plastic behaviour and n = 1 for Bingham plastic behaviour. For a shear thinning or shear thickening fluid the power law equation is specified as
For thixotropic fluids, mathematical models that are more complex than the models discussed so far are required. Thus, they are described by time-independent process viscosities normally fitted to the power law equation.
Taking viscosity measurements
Viscosity measurements are used extensively in the food industry.
It allows technologists to control the quality of raw materials when assessing the effects of changes in formulation, as well as the effect which processing conditions have on products and their development. When obtained from in-line systems or batch sample tests, viscosity measurements will help reduce ingredient costs and ensure batch-to-batch product consistency. General guidelines to taking viscosity measurements are at the end of this page.
The measuring equipment used is the viscometer. Two main types are rotational and capillary. Compared to capillary viscometers, rotational viscometers are more flexible and easier to use. On the other hand, at low viscosities and high shear rates, capillary viscometers have more accurate measurements than rotational viscometers.
Rotational viscometers are available as portable and stationary instruments. Portable types are operated manually, and usually come in a shock proof case equipped with all necessary accessories.
Usually, stationary installations automate measuring sequences and data evaluation with computer controls. The software includes possible fitting to a number of rheological models, plotting of flow curves and more.
Normally, a rotational viscometer is insufficient for a complete rheological analysis, which includes determining the structure breakdown in for instance, coconut milk yoghurt. For such analysis, a more sophisticated instrument called a rheometer is used. It operates with torsional vibration or oscillation rather than rotation. The fluid is also rheologically analysed without destroying its structure.
Viscosity in coconut milk
Viscosity in coconut milk is affected by added ingredients. For example, different manufacturers add different ingredients like starches, stabilizers and emulsifiers to coconut milk. As such, the viscosity data can vary widely among fluid foodstuffs. In particular, studies have shown that coconut milk, mixed with emulsifiers and subsequently pre-heated or homogenized exhibit pseudo-plastic, shear thinning behaviour over a 15-30% range of oil content (Tipvarakarnkoon, 2009). Viscosity of coconut milk is also affected by oil content, heat and homogenization (Table 10.2). Often, emulsifiers are added to homogenize coconut milk and extend the product’s shelf life.
Analysis of commercial samples at Tetra Pak’s in-house rheology lab (RheoLab) shows that, at a shear rate of 100s-1 between oil content of 17-25.1% at 30°C, the viscosity of coconut milk can range from as low as 8 mPas to as high as 260 mPas.
Increasing homogenization pressures can also increase viscosity. At higher pressures, the oil globules are broken into smaller and more numerous numbers, thereby increasing viscosity (Table 10.3).